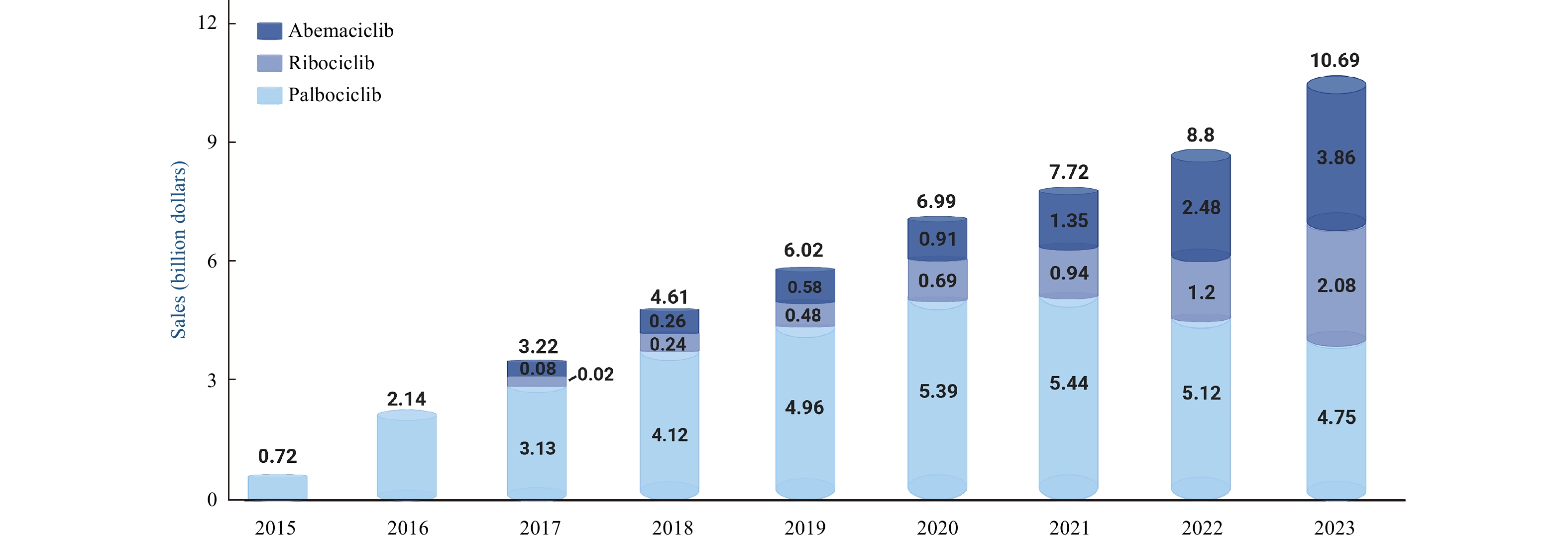
Citation: | Chen Weijiao, Zhuang Xujie, Chen Yuanyuan, Yang Huanaoyu, Shen Linhu, Feng Sikai, Min Wenjian, Yuan Kai, Yang Peng. Recent advances in regulating the cell cycle through inhibiting CDKs for cancer treatment[J]. Chinese Journal of Natural Medicines, 2025, 23(3): 286-298. DOI: 10.1016/S1875-5364(25)60846-6 |
The inhibition of cyclin-dependent kinases (CDKs) is considered a promising strategy for cancer treatment due to their role in cell cycle regulation. However, CDK inhibitors with no selectivity among CDK families have not been approved. A CDK inhibitor with high selectivity for CDK4/6 exhibited significant treatment effects on breast cancer and has become a heavy bomb on the market. Subsequently, resistance gradually decreased the efficacy of selective CDK4/6 inhibitors in breast cancer treatment. In this review, we first introduce the development of selective CDK4/6 inhibitors and then explain the role of CDK2 activation in inducing resistance to CDK4/6 inhibitors. Moreover, we focused on the development of CDK2/4/6 inhibitors and selective CDK2 inhibitors, which will aid in the discovery of novel CDK inhibitors targeting the cell cycle in the future.
Cyclin-dependent kinases (CDKs) play important roles in regulating the cell cycle, and reducing cyclin-dependent kinases (CDK) activity to slow cell cycle progression is considered an effective strategy for inhibiting cell proliferation and treating cancer 1, 2. However, the early CDK inhibitors that lacked selectivity among CDK families failed to enter the market due to their severe toxicity or poor efficacy 3. Subsequent research focused on developing selective CDK4/6 inhibitors, resulting in significant success 4, 5.
Palbociclib, the first approved selective CDK4/6 inhibitor, has significantly impacted the treatment landscape of hormone receptor (HR)+/human epidermal growth factor receptor 2 (HER2)–breast cancer treatment. The sales of CDK4/6 inhibitors have increased annually, reaching over
The cell cycle consists of interphase and the mitotic phase (M). The major function of interphase is to prepare the DNA and proteins that need to be used in the M stage, which are also separated into G1 (pre-DNA synthesis), S (DNA synthesis) and G2 (pre-division) 13, 14. Growing evidence has demonstrated that CDKs, including CDK1, 2, 4, 6, and 7, and their partner cyclins are pivotal regulators of cell cycle progression 15.
CDK4 and CDK6 are similar proteins that can be activated by cyclin D 16. The CDK4/6-cyclin D complex phosphorylates the retinoblastoma (RB) protein, leading to the release of E2F (Fig. 2) 17–19. This promotes the formation of the CDK2-cyclin E complex, which further phosphorylates RB and facilitates the transition from G1 to S phase 20–25. Subsequently, the CDK2-cyclin A complex continuously phosphorylates RB, driving the progression from S to G2 transition 26–28. Finally, the CDK1-cyclin A and CDK1-cyclin B complexes promote the initiation and the smooth progression of mitosis during the M phase (Fig. 2) 29–32. To prevent premature entry into mitosis, CDK1-related sites are phosphorylated by WEE1 and MYT1 kinases, which inhibit complex formation 33-37. Additionally, CDK7 regulates the cell cycle by activating CDKs 1, 2, 4, and 6 38–40.
Early-stage CDK inhibitors lacked selectivity within the CDK family (Fig. 3) 41–49. An-CDK inhibitors potentially suppress CDK subtypes essential for normal cell growth, leading to severe adverse effects 50–53. Moreover, the unclear anticancer mechanisms of pan-CDK inhibitors may result in inappropriate patient selection and reduced efficacy in clinical trials 43, 54–56. Although pan-CDK inhibitors exhibited potent anticancer activity in preclinical trials, many of them exhibited high toxicity or low efficacy in clinical trials 51, 57–60. Consequently, no pan-CDK inhibitors have successfully passed clinical trials and received market approval 1, 3.
Due to the disappointing outcomes of pan-CDK inhibitors clinical trials, the development of CDK inhibitors has focused on selectively inhibiting CDK4/6. Deletion of CDK4/6 did not affect the growth of normal cells; thus, targeting CDK4/6 might result in relatively low toxicity 4. Moreover, the selective inhibition of CDK4/6 exerted a single effect on the cell cycle to cause G1 arrest, and this clear anticancer mechanism could allow CDK4/6 inhibitors to find appropriate types of patients in clinical trials and demonstrate potent efficacy for cancer treatment 61. Currently, the selective CDK4/6 inhibitors palbociclib 5, 62, ribociclib 63, abemaciclib 64, and dalpiciclib 65 (Fig. 4) are approved for the treatment of HR+/HER2– breast cancer, and trilaciclib 66 (Fig. 4) is approved for the treatment of myelosuppression.
In HR+/HER2– breast cancer treatment, combining a CDK4/6 inhibitor with an aromatase inhibitor has demonstrated superior efficacy compared with aromatase inhibitor monotherapy. Consequently, this combination therapy has received regulatory approval for HR+/HER2– breast cancer 67–75. Furthermore, for patients experiencing disease progression after endocrine therapy, the combination of a CDK4/6 inhibitor with fulvestrant has shown enhanced therapeutic outcomes compared to fulvestrant alone. This selective CDK4/6 inhibitor/fulvestrant combination has subsequently been approved for treating HR+/HER2– breast cancer in patients who have experienced disease progression following endocrine therapy 76–82.
In contrast to palbociclib, ribociclib, and dalpiciclib, which require combination with endocrine therapy for breast cancer treatment, abemaciclib can also be utilized for treating breast cancer that has progressed following endocrine therapy 83. Furthermore, the combination of abemaciclib with endocrine therapy post-surgery in early breast cancer patients has demonstrated a significantly reduced recurrence rate compared to endocrine therapy alone. Consequently, in 2023, the Food and Drug Administration (FDA) approved the abemaciclib/endocrine therapy combination as an adjuvant treatment for HR+/HER2–, node-positive, early breast cancer patients at high risk of recurrence 84, 85.
In addition to breast cancer treatment, a selective CDK4/6 inhibitor could also be used to decrease myelosuppression induced by chemotherapy. Trilaciclib (Fig. 4), developed by G1 Therapeutics, received FDA approval in 2021 for the prevention of myelosuppression in patients with extensive-stage small cell lung cancer undergoing platinum/etoposide-containing or topotecan-containing regimens 66. Unlike palbociclib, ribociclib, abemaciclib, and dalpiciclib, which are administered orally, trilaciclib is delivered via intravenous injection. When administered before chemotherapy, trilaciclib rapidly induces G1 phase arrest in hematopoietic stem cells and progenitor cells, thereby protecting bone marrow cells from chemotherapy-induced damage 86–88.
Due to the extensive dysregulation of the cell cycle in various cancers, selective CDK4/6 inhibitors demonstrate potential for exhibiting potent efficacy across multiple cancer types. Currently, clinical trials of CDK4/6 inhibitors are being conducted to treat a wide range of malignancies, including acute leukemias, liver cancer, prostate cancer, pancreatic adenocarcinoma, liposarcoma, ovarian cancer, atypical neurofibromas, brain tumors and small-cell lung cancer (Table 1).
Drug | NCT number | Stage | Disease |
Palbociclib | NCT02310243 | I/II | Acute leukemias |
Palbociclib | NCT01356628 | II | Liver cancer |
Palbociclib | NCT02905318 | II | Prostate cancer |
Ribociclib/Everolimus | NCT02985125 | I/II | Pancreatic adenocarcinoma |
Ribociclib | NCT03096912 | II | Liposarcoma |
Ribociclib/Letrozole | NCT03673124 | II | Ovarian cancer |
Abemaciclib | NCT04750928 | I/II | Atypical neurofibromas |
Abemaciclib | NCT03220646 | II | Brain tumor |
Abemaciclib | NCT04967521 | III | Dedifferentiated liposarcoma |
Abemaciclib | NCT04010357 | II | Small-cell lung cancer |
CDK4/6 plays a pivotal role in the transition from the G1 to the S phase by promoting the phosphorylation of RB, which unleashes the activity of the transcription factor E2F. However, when the activity of CDK4/6 is inhibited, various compensatory mechanisms might be employed to bypass the inhibition. Among these mechanisms, one that has been extensively studied is the activation of CDK2. In this scenario, CDK2 is mobilized to continue the phosphorylation of RB, thereby releasing E2F and ensuring the progression of the cell cycle.
The efficacy of selective CDK4/6 inhibitors in breast cancer treatment has been significantly compromised by the development of resistance, prompting numerous studies to investigate the underlying mechanisms 8–10, 89–96. While various resistance mechanisms have been identified, each accounts for only a portion of the observed resistance. Among these, the activation of CDK2 has emerged as one of the most prominent and extensively studied mechanisms. Multiple investigations have demonstrated that CDK2 plays a crucial role in mediating resistance to CDK4/6 inhibitors in breast cancer (Fig. 5) 12, 95, 97, 98.
In ER+ breast cancer cells exposed to palbociclib, researchers have noted the regulation of cyclin D levels. Subsequently, cyclin D forms a complex with CDK2, known as the CDK2-cyclin D complex. This interaction leads to the restoration of RB phosphorylation, consequently diminishing palbociclib’s efficacy in inducing cell cycle arrest 99.
Phosphatase and tensin homolog (PTEN) is a tumor suppressor gene, which dephosphorylates phosphatidylinositol-3,4,5-trisphosphate (PIP3) and converts it to phosphatidylinositol-4,5-bisphosphate (PIP2), thereby negatively regulating the phosphatidylinositol 3-kinase (PI3K)/protein kinase B (AKT) signaling pathway. Inactivation of PTEN leads to an increase in PIP3 levels, which in turn activates the AKT signaling pathway, promoting cell growth and survival and increasing the risk of tumor development. The absence of PTEN was observed in ER+ breast cancer patients who were resistant to the ribociclib/letrozole combination. Loss of PTEN upregulated AKT activity and promoted resistance to CDK4/6 inhibitors in ER+ breast cancer cells. The combination of an AKT inhibitor with ribociclib displayed antitumor activity against PTEN-deficient breast cancer. Further mechanistic studies indicated that the activation of AKT triggers the exocytosis of p27 from the nucleus and subsequently leads to a reduction in p27 activity. Subsequently, the activity of downstream CDK2 and CDK4 increased, which ultimately induced resistance to CDK4/6 inhibitors 92, 100, 101.
Pyruvate dehydrogenase kinase 1 (PDK1) is the pivotal node in the PI3K/AKT pathway, and the activation of PDK1 can activate AKT to promote cell proliferation and inhibit apoptosis. Knockdown of PDK1 increased the antiproliferative activity of ribociclib against ER + breast cancer cells, and the combination of a PDK1 inhibitor with ribociclib also had synergistic effects on ER + breast cancer cells. Elevated levels of PDK1 expression were detected in ribociclib-resistant breast cancer cells, resulting in AKT pathway activation. This upregulated the expression of cyclin A and cyclin E and promoted the activation of CDK2, ultimately contributing to the development of drug resistance. Both PDK1 inhibitors and CDK2 inhibitors can increase the sensitivity of resistant cells to ribociclib 102.
Clinical trials have provided substantial evidence for the critical role of the CDK2-cyclin E complex in the emergence of CDK4/6 inhibitor resistance. Amplification of CCNE was observed in ER+ breast cancer patients treated with a CDK4/6 inhibitor 92, 103. In a cohort of ER+ breast cancer patients treated with palbociclib/letrozole or palbociclib/fulvestrant, PFS significantly decreased when proteolytically cleaved cytoplasmic cyclin E in tumor tissue was detected 104. Notably, the PALOMA3 trial showed that patients with lower levels of cyclin E exhibited better treatment effects than those with higher levels of cyclin E when they received palbociclib/fulvestrant 103.
In summary, these studies demonstrated that CDK2 plays a critical role in maintaining normal cell cycle progression in CDK4/6 inhibitor-resistant breast cancer cells. Furthermore, the combination of CDK2 inhibition, either through knockdown or targeted inhibitors, with CDK4/6 inhibitors significantly impeded the proliferation of resistant cells. Recognizing the pivotal role of CDK2 in CDK4/6 inhibitor resistance, researchers have developed CDK2/4/6 inhibitors and selective CDK2 inhibitors as potential strategies to overcome this resistance.
Several CDK2/4/6 inhibitors, including PF-06873600, NUV-422, RGT-419B, and SCR-8079 (Table 2), have been developed. PF-06873600, developed by Pfizer, was the first CDK2/4/6 inhibitor which come into clinical trials 105. Through a mobility shift assay, Pfizer discovered palbociclib derivative 1a (Fig. 6) with CDK2 inhibitory activity (Ki = 5.9 nmol·L−1). The crystal structure of 1a with CDK2 revealed that the introduced piperidine sulfonamide motif formed hydrogen bond interactions with Asp86 and Lys89. The removal of the methyl group at the C'5 position and acetyl group at the C'6 position from 1a afforded 1b (Fig. 6), which improved the inhibitory activity against CDK2 (Ki = 0.71 nmol·L−1) and selectivity over CDK1 (16.5-fold). The introduction of a methyl group to the cyclopentyl ring at the N'8 position generated 1c (Fig. 6), which exhibited more potent CDK2 inhibitory activity (Ki = 0.09 nmol·L−1) and higher selectivity over CDK1 (26.7-fold). However, human liver microsome (HLM) clearance was also significantly increased (Clint > 482 μL·min−1·mg−1) 106–109. Further optimization of the cyclopentyl ring provided 1d (Fig. 6), which retained great CDK2 potency (Ki = 0.2 nmol·L−1), high CDK1 selectivity (22-fold) and increased HLM stability (Clint = 36.4 μL·min−1·mg−1). The addition of the methyl group at the C'5 position (1e) increased selectivity over CDK1 (45.8-fold) but decreased HLM stability (Clint = 67.4 μL·min−1·mg−1). Finally, the replacement of methyl with difluoromethyl provided PF-06873600, which exhibited obviously improved HLM stability (Clint < 10.8 μL·min−1·mg−1) and retained potent CDK2 inhibitory activity (Ki = 0.13 nmol·L−1) and high CDK1 selectivity (34.6-fold) 105.
Name | Structure | Company | Stage |
PF-06873600 | ![]() |
Pfizer | Discontinued |
NUV-422 | ![]() |
Nuvation Bio | Discontinued |
RGT-419B | Not available | Regor | Phase I |
SCR-8079 | Not available | Simcere | Preclinical |
The crystal structure analysis of PF-06873600 bound to CDK2 revealed specific interactions. The methylsulfonyl groups' oxygen atoms formed hydrogen bonds with Asp86 and Lys89 (Fig. 7). the 2-aminopyrimidine moiety of PF-06873600 established two characteristic hydrogen bonds with the hinge region Leu83. At the N'8 position, the cyclopentyl group occupied the lipophilic pocket, while the hydroxyl group's oxygen atom formed a hydrogen bond with Gln131. The difluoromethyl group at the C'5 position formed van der Waals interactions with the gatekeeper Phe80 (Fig. 7) 105.
Preclinical studies demonstrated that PF-06873600 exhibited inhibitory activity against hormone receptor-positive (HR+) breast cancer and could potentially overcome resistance to cyclin-dependent kinase 4/6 (CDK4/6) inhibitors in HR+ breast cancer 110. In addition, palbociclib did not significantly affect CCNE1-amplified cancers 111, and PF-06873600 potently inhibited these cancers. However, the clinical trial of PF-06873600 was discontinued in November 2022.
CDK2/4/6 inhibitor NUV-422 was granted orphan drug designation by the FDA for malignant glioma treatment in 2021. The indications for NUV-422 in clinical trials included glioma, HR+/HER2− breast cancer, and metastatic castration-resistant prostate cancer. The partial clinical trial of NUV-422 was suspended by the FDA due to the emergence of uveitis in June 2022, and Nuvation Bio discontinued the clinical trial of NUV-422 based on the internal risk-benefit analysis in August 2022.
RGT-419B, developed by Regor Therapeutics, is currently undergoing clinical trials for the treatment of HR+/HER2- breast cancer and other solid tumors in Chinese patients (NCT06299124), as well as HR+/HER2- breast cancer with resistance to CDK4/6 inhibitors (NCT05304962). Furthermore, SCR-8079, a CDK2/4/6 inhibitor developed by Simcere Pharmaceutical, demonstrated potent inhibitory activity against CDK4/6 inhibitor-resistant cells in preclinical studies. This compound significantly inhibited the growth of OVCAR3 and MCF-7 xenograft tumors 112-117.
Selective CDK2 inhibitors have been a research hotspot in recent years, and a number of selective CDK2 inhibitors, including PF07104091, BLU-222, INCB123667, INX-315, ARTS-021, AZD8421, NKT-3447, and BG-
Name | Structure | Company | Datea |
PF07104091 | ![]() |
Pfizer | 2020-09-16 |
BLU-222 | ![]() |
Blueprint Medicine | 2022-04-07 |
INCB123667 | Not available | Incyte | 2022-07-05 |
INX-315 | ![]() |
Incyclix Bio | 2023-03-28 |
ARTS-021 | Not available | Allorion Therapeutics | 2023-08-30 |
AZD8421 | ![]() |
AstraZeneca | 2023-12-05 |
NKT-3447 | Not available | NiKang Therapeutics | 2024-02-23 |
BG-68501 | Not available | Beigene | 2024-02-28 |
aThe actual date on which the first participant was enrolled in the clinical study. |
PF07104091 was the first selective CDK2 inhibitor to enter clinical trials. The Phase I trial of PF07104091 (NCT05431153) was completed, demonstrating that PF07104091 as a single agent exhibited tolerability and anticancer activity in breast cancer patients who had developed resistance to CDK4/6 inhibitors 118.
The VELA trial of BLU-222 demonstrated that transient visual adverse events occurred in five patients (19%) by January 2023 119, and the partial VELA trial was put aside by FDA due to visual adverse events in February 2023. The patients with visual adverse events all recovered when the dose was interrupted or reduced, and further ophthalmologic examinations confirmed that no abnormal findings were observed in the patients after treatment. As a result, this clinical hold on the VELA trial was canceled in March 2023.
INX-315 was developed by Incyclix Bio through structural optimization of trilaciclib (Fig. 8) 120. The removal of the lactam carbonyl and replacement of the pyridine-piperazine motif with benzyl sulfonamide provided 2a (Fig. 8), which exhibited significantly improved CDK1 and CDK2 inhibitory activity, but the HLM clearance of 2a was extremely high, with a half-life of less than 10 min. The optimization of the tricyclic core generated 2b (Fig. 8), which exhibited reduced inhibition of CDKs 1, 2, 4, 6, and 9 and slightly increased HLM stability. Further modification of the tricyclic core provided INX-315 (Fig. 8), which displayed significantly improved CDK2 potency, selectivity toward CDKs 1, 4, 6, and 9, and metabolic stability 115. The predicted binding model of INX-315 with CDK2 indicated that the sulfonamide moiety formed two hydrogen bonds with Asp86 and one hydrogen bond with Lys89. In addition, the 2-aminopyrimidine formed hydrogen bonds with Leu83, and the tricyclic core formed one hydrogen bond with Asp145 (Fig. 9) 121.
INX-315 demonstrated the ability to inhibit RB phosphorylation, leading to cell cycle arrest and promotion of therapy-induced senescence (TIS). This mechanism resulted in tumor growth inhibition in CCNE1-amplified cancers. In CDK4/6 inhibitor-resistant breast cancer, cell proliferation relied on both CDK4/6 and CDK2. Consequently, combining INX-315 with a CDK4/6 inhibitor exhibited optimal control of proliferation. Additionally, this combination delayed resistance development through enhanced suppression of E2F targets. It is noteworthy that the senescence induced by CDK4/6 or CDK2 inhibitors is reversible and dynamic. CDK4/6 inhibitors remodeled chromatin to induce senescence, and upon resistance development, the chromatin could partially revert to a pre-senescent state. Subsequently, the addition of INX-315 could reverse chromatin to a senescence architecture and reinstate TIS 122.
The structure of INCB123667, developed by Incyte, has not been revealed, but Incyte reported two kinds of selective CDK2 inhibitors, 3 (Fig. 10) and 4 (Fig.11) 123,124. Hit 3a (Fig. 10) was identified as a CDK2 inhibitor with an IC50 value of 431 nmol·L−1 through a high-throughput screen (HTS). Merging pyrimidine and pyrazole scaffolds provided 3b (Fig. 10), which showed increased CDK2 inhibitory activity, but the potency of 3b was lost in human whole blood (hWB) 125–127. The addition of a methyl group to the sulfonamide and the modification of the lactam substituent (3c, Fig. 10) resolved the issues of hWB potency loss and increased selectivity over CDKs 1, 4, and 6. The introduction of polar substituents to the lactam nitrogen generated 3d (Fig. 10), which exhibited increased HLM stability (hCl = 0.8 L·h−1·kg−1). However, the sulfonamide demethylation of 3d resulted in high in vivo clearance 128, 129. To resolve this issue, protons were replaced with deuterium to generate 3 (Fig. 10) 130, which displayed obviously decreased sulfonamide demethylation and increased stability in HLM 123.
The hit 4a (Fig. 11) was also discovered through the HTS of Incyte’s in-house compound collection and exhibited medium CDK2 inhibitory activity (IC50 = 607 nmol·L−1) and potent JAK inhibitory activity (IC50 < 1 nmol·L−1) 131-133. The preliminary structural optimization generated 4b (Fig. 11), which showed significantly improved inhibitory activity against CDK2 (IC50 = 16 nmol·L−1) and selectivity over JAK2 (IC50 >
The crystal structure of 4 with CDK2 revealed that the two oxygen atoms of cyclopropylsulfonyl formed hydrogen bond interactions with Asp86 and Lys89, and the 2-aminopyrimidine moiety formed two hydrogen bonds with Leu83 (Fig. 12). The triffuoromethyl of pyrimidine was projected toward the gatekeeper Phe80. In addition, the hydroxyl group formed one hydrogen bond with Asp145 (Fig. 12). 4 exhibited great selectivity among more than 50 kinases and no obvious inhibition against multiple cytochrome P450 isoforms or hERG channels, and pharmacokinetic studies in the cyno variety indicated that 4 displayed low clearance, great exposure, and favorable oral bioavailability. Moreover, 4 significantly inhibited RB phosphorylation and tumor growth in the CCNE1-amplified OVCAR3 tumors 124.
The discovery of CDKs’ role in cell cycle regulation has prompted extensive research into CDK inhibitors as potential cancer treatments 137–156. Approved CDK inhibitors, particularly those selectively targeting CDK4/6, have demonstrated significant efficacy and manageable toxicity, becoming the standard first-line therapy for breast cancer 157-163. However, the development of resistance to CDK4/6 inhibitors poses a substantial challenge to treatment efficacy in breast cancer. Research into this resistance has identified CDK2 activation as a key factor. In response, researchers have developed CDK2/4/6 inhibitors and selective CDK2 inhibitors to combat CDK4/6 inhibitor resistance, offering a promising approach for treating CCNE1-amplified cancers 164-171.
The progression of CDK2/4/6 inhibitors through clinical trials has encountered challenges, with trials for PF-06873600 and NUV-422 being terminated in 2022. In contrast, selective CDK2 inhibitors have garnered increasing interest, with numerous such inhibitors entering clinical trials over the past two years. Compared to CDK2/4/6 inhibitors, selective CDK2 inhibitors potentially offer a safer treatment option for CCNE1-amplified cancers by eliminating the toxicity associated with CDK4/6 inhibition. In addition, the combination of a CDK2 inhibitor and a CDK4/6 inhibitor was more flexible than a CDK2/4/6 inhibitor in CDK4/6 inhibitor-resistant breast cancer. By optimizing the dosage of the CDK2 inhibitor and CDK4/6 inhibitor, a treatment schedule with potent efficacy and favorable toxicity was likely to be obtained 172-181.
In addition to targeting CDK2, inhibiting CDK7 also shows promise in overcoming CDK4/6 inhibitor resistance. Clinical trials have demonstrated the efficacy of combining the CDK7 inhibitor samuraciclib with fulvestrant in patients resistant to CDK4/6 inhibitors 182-185. Notably, resistance mechanisms differ among CDK4/6 inhibitors, such as palbociclib and abemaciclib, with abemaciclib exhibiting significant effects on palbociclib-resistant breast cancer. This underscores the continued necessity for developing novel CDK4/6 inhibitors to address resistance to existing inhibitors. The emerging field of protein degradation in cancer therapy presents another avenue for overcoming resistance, particularly through CDK4/6 degradation, warranting further investigation. While most resistance mechanism studies have focused on CDK4/6 inhibitor monotherapy, patients typically receive combination therapy with endocrine treatments. Future research should emphasize resistance mechanisms in combination therapy to enhance clinical applications. In addition, the most mechanism studies of CDK4/6 inhibitors resistance focused the monotherapy of CDK4/6 inhibitors, but patients often received the combination of CDK4/6 inhibitors and endocrine therapy. Therefore, more mechanism studies should focus on the resistance to combination therapy, which might bring the better clinical application. Furthermore, many CDK inhibitors were derived from natural products, such as flavoalkaloids flavopiridol and riviciclib, indirubin derivatives AG-024322. Meanwhile, many natural products, such as icaritin, pristimerin, asparanin A, licochalcone B, juglone, could regulate activity or expression of CDKs and exhibit potent anticancer activity. Further exploration of natural products may yield novel CDK inhibitors and address limitations of existing inhibitors. In conclusion, the development of CDK inhibitors targeting the cell cycle remains a crucial strategy in cancer treatment 186-212.
These authors have no conflict of interest to declare.
[1] |
Asghar U, Witkiewicz AK, Turner NC, et al. The history and future of targeting cyclin-dependent kinases in cancer therapy. Nat Rev Drug Discov. 2015;14:130-146. https://doi.org/10.1038/nrd4504.
|
[2] |
Malumbres M, Barbacid M. Cell cycle, CDKs and cancer: a changing paradigm. Nat Rev Cancer. 2009;9:153-166. https://doi.org/10.1038/nrc2602.
|
[3] |
Zhang M, Zhang L, Hei R, et al. CDK inhibitors in cancer therapy, an overview of recent development. Am J Cancer Res. 2021;11:1913-1935.
|
[4] |
O’Leary B, Finn RS, Turner NC. Treating cancer with selective CDK4/6 inhibitors. Nat Rev Clin Oncol. 2016;13:417-430. https://doi.org/10.1038/nrclinonc.2016.26.
|
[5] |
Dhillon S. Palbociclib: first global approval. Drugs. 2015;75:543-551. https://doi.org/10.1007/s40265-015-0379-9.
|
[6] |
Goel S, Bergholz JS, Zhao JJ. Targeting CDK4 and CDK6 in cancer. Nat Rev Cancer. 2022;22:356-372. https://doi.org/10.1038/s41568-022-00456-3.
|
[7] |
Morrison L, Loibl S, Turner NC. The CDK4/6 inhibitor revolution — a game-changing era for breast cancer treatment. Nat Rev Clin Oncol. 2024;21:89-105. https://doi.org/10.1038/s41571-023-00840-4.
|
[8] |
Cetin B, Wabl CA, Gumusay O. CDK4/6 inhibitors: mechanisms of resistance and potential biomarkers of responsiveness in breast cancer. Future Oncol. 2022;18:1143-1157. https://doi.org/10.2217/fon-2021-0842.
|
[9] |
Huang J, Zheng L, Sun Z, et al. CDK4/6 inhibitor resistance mechanisms and treatment strategies (Review). Int J Mol Med. 2022;50:128. https://doi.org/10.3892/ijmm.2022.5184.
|
[10] |
Lloyd MR, Spring LM, Bardia A, et al. Mechanisms of resistance to CDK4/6 blockade in advanced hormone receptor–positive, HER2-negative breast cancer and emerging therapeutic opportunities. Clin Cancer Res. 2022;28:821-830. https://doi.org/10.1158/1078-0432.CCR-21-2947.
|
[11] |
Fassl A, Geng Y, Sicinski P. CDK4 and CDK6 kinases: From basic science to cancer therapy. Science. 2022;375:eabc1495. https://doi.org/10.1126/science.abc1495.
|
[12] |
Gerosa R, De Sanctis R, Jacobs F, et al. Cyclin-dependent kinase 2 (CDK2) inhibitors and others novel CDK inhibitors (CDKi) in breast cancer: clinical trials, current impact, and future directions. Crit Rev Oncol Hemat. 2024;196:104324. https://doi.org/10.1016/j.critrevonc.2024.104324.
|
[13] |
Nurse P, Masui Y, Hartwell L. Understanding the cell cycle. Nat Med. 1998;4:1103-1106. https://doi.org/10.1038/2594.
|
[14] |
Bai J, Li Y, Zhang G. Cell cycle regulation and anticancer drug discovery. Cancer Biol Med. 2017;14:348-362. https://doi.org/10.20892/j.issn.2095-3941.2017.0033.
|
[15] |
Nurse P. Cyclin dependent kinases and cell cycle control (nobel lecture). Chembiochem. 2002;3:596-603.3.0.CO;2-U"> https://doi.org/10.1002/1439-7633(20020703)3:7<596::AID-CBIC596>3.0.CO;2-U. doi: 10.1002/1439-7633(20020703)3:7<596::AID-CBIC596>3.0.CO;2-U
|
[16] |
Pavletich NP. Mechanisms of cyclin-dependent kinase regulation: structures of Cdks, their cyclin activators, and Cip and INK4 inhibitors. J Mol Biol. 1999;287:821-828. https://doi.org/10.1006/jmbi.1999.2640.
|
[17] |
Sherr CJ. D-type cyclins. Trends Biochem Sci. 1995;20:187-190. https://doi.org/10.1016/S0968-0004(00)89005-2.
|
[18] |
Diehl JA. Cycling to cancer with cyclin D1. Cancer Biol Ther. 2002;1:226-231. https://doi.org/10.4161/cbt.72.
|
[19] |
Baldin V, Lukas J, Marcote MJ, et al. Cyclin D1 is a nuclear protein required for cell cycle progression in G1. Genes Dev. 1993;7:812-821. https://doi.org/10.1101/gad.7.5.812.
|
[20] |
Goodrich DW, Wang NP, Qian YW, et al. The retinoblastoma gene product regulates progression through the G1 phase of the cell cycle. Cell. 1991;67:293-302. https://doi.org/10.1016/0092-8674(91)90181-W.
|
[21] |
Harbour JW, Luo RX, Santi AD, et al. Cdk phosphorylation triggers sequential intramolecular interactions that progressively block Rb functions as cells move through G1. Cell. 1999;98:859-869. https://doi.org/10.1016/S0092-8674(00)81519-6.
|
[22] |
Pagano M, Draetta G, Jansen-Dürr P. Association of CDK2 kinase with the transcription factor E2F during S phase. Science. 1992;255:1144-1147. https://doi.org/10.1126/science.1312258.
|
[23] |
Devoto SH, Mudryj M, Pines J, et al. A cyclin A-protein kinase complex possesses sequence-specific DNA binding activity: p33cdk2 is a component of the E2F-cyclin A complex. Cell. 1992;68:167-176. https://doi.org/10.1016/0092-8674(92)90215-X.
|
[24] |
Dubey R, Makhija R, Sharma A, et al. Unveiling the promise of pyrimidine-modified CDK inhibitors in cancer treatment. Bioorg Chem. 2024;149:107508. https://doi.org/10.1016/j.bioorg.2024.107508.
|
[25] |
Cavalu S, Abdelhamid AM, Saber S, et al. Cell cycle machinery in oncology: A comprehensive review of therapeutic targets. FASEB J. 2024;38:e23734. https://doi.org/10.1096/fj.202400769R.
|
[26] |
Peng C, Zeng W, Su J, et al. Cyclin-dependent kinase 2 (CDK2) is a key mediator for EGF-induced cell transformation mediated through the ELK4/c-Fos signaling pathway. Oncogene. 2016;35:1170-1179. https://doi.org/10.1038/onc.2015.175.
|
[27] |
Ding L, Cao J, Lin W, et al. The Roles of cyclin-dependent kinases in cell-cycle progression and therapeutic strategies in human breast cancer. Int J Mol Sci. 2020;21:1960. https://doi.org/10.3390/ijms21061960.
|
[28] |
Lashen A, Alqahtani S, Shoqafi A, et al. Clinicopathological significance of cyclin-dependent kinase 2 (CDK2) in ductal carcinoma in situ and early-stage invasive breast cancers. Int J Mol Sci. 2024;25:5053. https://doi.org/10.3390/ijms25095053.
|
[29] |
Malumbres M. Cyclin-dependent kinases. Genome Biol. 2014;15:122. https://doi.org/10.1186/gb4184.
|
[30] |
Massacci G, Perfetto L, Sacco F. The cyclin-dependent kinase 1: more than a cell cycle regulator. Br J Cancer. 2023;129:1707-1716. https://doi.org/10.1038/s41416-023-02468-8.
|
[31] |
Diril MK, Ratnacaram CK, Padmakumar VC, et al. Cyclin-dependent kinase 1 (CDK1) is essential for cell division and suppression of DNA re-replication but not for liver regeneration. Proc Natl Acad Sci USA. 2012;109:3826-3831. https://doi.org/10.1073/pnas.1115201109.
|
[32] |
Lima JT, Ferreira JG. Mechanobiology of the nucleus during the G2-M transition. Nucleus. 2024;15:2330947. https://doi.org/10.1080/19491034.2024.2330947.
|
[33] |
Parker LL, Piwnica-Worms H. Inactivation of the p34cdc2-cyclin B complex by the human WEE1 tyrosine kinase. Science. 1992;257:1955-1957. https://doi.org/10.1126/science.1384126.
|
[34] |
Knight GL, Turnell AS, Roberts S. Role for WEE1 in inhibition of G2-to-M transition through the cooperation of distinct human papillomavirus type 1 E4 proteins. J Virol. 2006;80:7416-7426. https://doi.org/10.1128/JVI.00196-06.
|
[35] |
Chow JPH, Poon RYC. The CDK1 inhibitory kinase Myt1 in DNA damage checkpoint recovery. Oncogene. 2013;32:4778-4788. https://doi.org/10.1038/onc.2012.504.
|
[36] |
Liu F, Stanton JJ, Wu Z, et al. The human Myt1 kinase preferentially phosphorylates Cdc2 on threonine 14 and localizes to the endoplasmic reticulum and Golgi complex. Mol Cell Biol. 1997;17:571-583. https://doi.org/10.1128/MCB.17.2.571.
|
[37] |
Yan J, Zhuang L, Wang Y, et al. Inhibitors of cell cycle checkpoint target Wee1 kinase – A patent review (2003–2022). Expert Opin Ther Pat. 2022;32:1217-1244. https://doi.org/10.1080/13543776.2022.2166827.
|
[38] |
Kolloch L, Kreinest T, Meisterernst M, et al. Control of expression of key cell cycle enzymes drives cell line-specific functions of CDK7 in human PDAC cells. Int J Mol Sci. 2022;23:812. https://doi.org/10.3390/ijms23020812.
|
[39] |
Wilson GA, Vuina K, Sava G, et al. Active growth signaling promotes senescence and cancer cell sensitivity to CDK7 inhibition. Mol Cell. 2023;83:4078-4092. e6.
|
[40] |
Sava GP, Fan H, Coombes RC, et al. CDK7 inhibitors as anticancer drugs. Cancer Metastasis Rev. 2020;39:805-823. https://doi.org/10.1007/s10555-020-09885-8.
|
[41] |
Airik R, Airik M, Schueler M, et al. Roscovitine blocks collecting duct cyst growth in Cep164-deficient kidneys. Kidney Int. 2019;96:320-326. https://doi.org/10.1016/j.kint.2019.04.014.
|
[42] |
Meijer L, Hery-Arnaud G, Leven C, et al. Safety and pharmacokinetics of Roscovitine (Seliciclib) in cystic fibrosis patients chronically infected with Pseudomonas aeruginosa, a randomized, placebo-controlled study. J Cyst Fibros. 2022;21:529-536. https://doi.org/10.1016/j.jcf.2021.10.013.
|
[43] |
Bose P, Simmons GL, Grant S. Cyclin-dependent kinase inhibitor therapy for hematologic malignancies. Expert Opin Investig Drugs. 2013;22:723-738. https://doi.org/10.1517/13543784.2013.789859.
|
[44] |
Parry D, Guzi T, Shanahan F, et al. Dinaciclib (SCH 727965), a novel and potent cyclin-dependent kinase inhibitor. Mol Cancer Ther. 2010;9:2344-2353. https://doi.org/10.1158/1535-7163.MCT-10-0324.
|
[45] |
Sedlacek H, Czech J, Naik R, et al. Flavopiridol (L86 8275; NSC 649890), a new kinase inhibitor for tumor therapy. Int J Oncol. 1996;9:1143-1168.
|
[46] |
Joshi KS, Rathos MJ, Joshi RD, et al. In vitro antitumor properties of a novel cyclin-dependent kinase inhibitor, P276-00. Mol Cancer Ther. 2007;6:918-925. https://doi.org/10.1158/1535-7163.MCT-06-0613.
|
[47] |
Byth KF, Thomas A, Hughes G, et al. AZD5438, a potent oral inhibitor of cyclin-dependent kinases 1, 2, and 9, leads to pharmacodynamic changes and potent antitumor effects in human tumor xenografts. Mol Cancer Ther. 2009;8:1856-1866. https://doi.org/10.1158/1535-7163.MCT-08-0836.
|
[48] |
Tong W-G, Chen R, Plunkett W, et al. Phase I and pharmacologic study of SNS-032, a potent and selective Cdk2, 7, and 9 inhibitor, in patients with advanced chronic lymphocytic leukemia and multiple myeloma. J Clin Oncol. 2010;28:3015-3022. https://doi.org/10.1200/JCO.2009.26.1347.
|
[49] |
DePinto W, Chu XJ, Yin X, et al. In vitro and in vivo activity of R547: a potent and selective cyclin-dependent kinase inhibitor currently in phase I clinical trials. Mol Cancer Ther. 2006;5:2644-2658.
|
[50] |
Reck M, Horn L, Novello S, et al. Phase II study of roniciclib in combination with cisplatin/etoposide or carboplatin/etoposide as first-line therapy in patients with extensive-disease small cell lung cancer. J Thorac Oncol. 2019;14:701-711. https://doi.org/10.1016/j.jtho.2019.01.010.
|
[51] |
Boss DS, Schwartz GK, Middleton MR, et al. Safety, tolerability, pharmacokinetics and pharmacodynamics of the oral cyclin-dependent kinase inhibitor AZD5438 when administered at intermittent and continuous dosing schedules in patients with advanced solid tumours. Ann Oncol. 2010;21:884-894. https://doi.org/10.1093/annonc/mdp377.
|
[52] |
Kouroukis CT, Belch A, Crump M, et al. Flavopiridol in untreated or relapsed mantle-cell lymphoma: results of a phase II study of the National Cancer Institute of Canada Clinical Trials Group. J Clin Oncol. 2003;21:1740-1745. https://doi.org/10.1200/JCO.2003.09.057.
|
[53] |
Le Tourneau C, Faivre S, Laurence V, et al. Phase I evaluation of seliciclib (R-roscovitine), a novel oral cyclin-dependent kinase inhibitor, in patients with advanced malignancies. Eur J Cancer. 2010;46:3243-3250. https://doi.org/10.1016/j.ejca.2010.08.001.
|
[54] |
Stephenson JJ, Nemunaitis J, Joy AA, et al. Randomized phase 2 study of the cyclin-dependent kinase inhibitor dinaciclib (MK-7965) versus erlotinib in patients with non-small cell lung cancer. Lung Cancer. 2014;83:219-223. https://doi.org/10.1016/j.lungcan.2013.11.020.
|
[55] |
Mita MM, Joy AA, Mita A, et al. Randomized phase II trial of the cyclin-dependent kinase inhibitor dinaciclib (MK-7965) versus capecitabine in patients with advanced breast cancer. Clin Breast Cancer. 2014;14:169-176. https://doi.org/10.1016/j.clbc.2013.10.016.
|
[56] |
Cassaday RD, Goy A, Advani S, et al. A phase II, single-arm, open-label, multicenter study to evaluate the efficacy and safety of P276-00, a cyclin-dependent kinase inhibitor, in patients with relapsed or refractory mantle cell lymphoma. Clin Lymphoma Myeloma Leuk. 2015;15:392-397. https://doi.org/10.1016/j.clml.2015.02.021.
|
[57] |
Shapiro GI. Cyclin-dependent kinase pathways as targets for cancer treatment. J Clin Oncol. 2006;24:1770-1783. https://doi.org/10.1200/JCO.2005.03.7689.
|
[58] |
Nemunaitis JJ, Small KA, Kirschmeier P, et al. A first-in-human, phase 1, dose-escalation study of dinaciclib, a novel cyclin-dependent kinase inhibitor, administered weekly in subjects with advanced malignancies. J Transl Med. 2013;11:259. https://doi.org/10.1186/1479-5876-11-259.
|
[59] |
Ghia P, Scarfò L, Perez S, et al. Efficacy and safety of dinaciclib vs ofatumumab in patients with relapsed/refractory chronic lymphocytic leukemia. Blood. 2017;129:1876-1878. https://doi.org/10.1182/blood-2016-10-748210.
|
[60] |
Raje N, Hideshima T, Mukherjee S, et al. Preclinical activity of P276-00, a novel small-molecule cyclin-dependent kinase inhibitor in the therapy of multiple myeloma. Leukemia. 2009;23:961-970. https://doi.org/10.1038/leu.2008.378.
|
[61] |
Corona SP, Ravelli A, Cretella D, et al. CDK4/6 inhibitors in HER2-positive breast cancer. Crit Rev Oncol Hemat. 2017;112:208-214. https://doi.org/10.1016/j.critrevonc.2017.02.022.
|
[62] |
Lynce F, Shajahan-Haq AN, Swain SM. CDK4/6 inhibitors in breast cancer therapy: Current practice and future opportunities. Pharmacol Therapeut. 2018;191:65-73. https://doi.org/10.1016/j.pharmthera.2018.06.008.
|
[63] |
Syed YY. Ribociclib: First global approval. Drugs. 2017;77:799-807. https://doi.org/10.1007/s40265-017-0742-0.
|
[64] |
Kim ES. Abemaciclib: First global approval. Drugs. 2017;77:2063-2070. https://doi.org/10.1007/s40265-017-0840-z.
|
[65] |
Chen X, Shen K. Dalpiciclib in advanced breast cancer. Lancet Oncol. 2023;24:578-579. https://doi.org/10.1016/S1470-2045(23)00228-0.
|
[66] |
Dhillon S. Trilaciclib: first approval. Drugs. 2021;81:867-874. https://doi.org/10.1007/s40265-021-01508-y.
|
[67] |
Finn RS, Martin M, Rugo HS, et al. Palbociclib and letrozole in advanced breast cancer. N Engl J Med. 2016;375:1925-1936. https://doi.org/10.1056/NEJMoa1607303.
|
[68] |
Turner NC, Ro J, André F, et al. Palbociclib in hormone-receptor–positive advanced breast cancer. N Engl J Med. 2015;373:209-219. https://doi.org/10.1056/NEJMoa1505270.
|
[69] |
Rugo HS, Finn RS, Diéras V, et al. Palbociclib plus letrozole as first-line therapy in estrogen receptor-positive/human epidermal growth factor receptor 2-negative advanced breast cancer with extended follow-up. Breast Cancer Res Treat. 2019;174:719-729. https://doi.org/10.1007/s10549-018-05125-4.
|
[70] |
Hortobagyi GN, Stemmer SM, Burris HA, et al. Ribociclib as first-line therapy for HR-Positive, advanced breast cancer. N Engl J Med. 2016;375:1738-1748. https://doi.org/10.1056/NEJMoa1609709.
|
[71] |
Hortobagyi GN, Stemmer SM, Burris HA, et al. Updated results from MONALEESA-2, a phase III trial of first-line ribociclib plus letrozole versus placebo plus letrozole in hormone receptor-positive, HER2-negative advanced breast cancer. Ann Oncol. 2018;29:1541-1547. https://doi.org/10.1093/annonc/mdy155.
|
[72] |
Hortobagyi GN, Stemmer SM, Burris HA, et al. Overall survival with ribociclib plus letrozole in advanced breast cancer. N Engl J Med. 2022;386:942-950. https://doi.org/10.1056/NEJMoa2114663.
|
[73] |
Goetz MP, Toi M, Campone M, et al. MONARCH 3: abemaciclib as initial therapy for advanced breast cancer. J Clin Oncol. 2017;35:3638-3646. https://doi.org/10.1200/JCO.2017.75.6155.
|
[74] |
Johnston S, Martin M, Di Leo A, et al. MONARCH 3 final PFS: a randomized study of abemaciclib as initial therapy for advanced breast cancer. NPJ Breast Cancer. 2019;5:5. https://doi.org/10.1038/s41523-018-0097-z.
|
[75] |
Zhang P, Zhang Q, Tong Z, et al. Dalpiciclib plus letrozole or anastrozole versus placebo plus letrozole or anastrozole as first-line treatment in patients with hormone receptor-positive, HER2-negative advanced breast cancer (DAWNA-2): a multicentre, randomised, double-blind, placebo-controlled, phase 3 trial. Lancet Oncol. 2023;24:646-657. https://doi.org/10.1016/S1470-2045(23)00172-9.
|
[76] |
Cristofanilli M, Turner NC, Bondarenko I, et al. Fulvestrant plus palbociclib versus fulvestrant plus placebo for treatment of hormone-receptor-positive, HER2-negative metastatic breast cancer that progressed on previous endocrine therapy (PALOMA-3): final analysis of the multicentre, double-blind, phase 3 randomised controlled trial. Lancet Oncol. 2016;17:425-439. https://doi.org/10.1016/S1470-2045(15)00613-0.
|
[77] |
Cristofanilli M, Rugo HS, Im S-A, et al. Overall survival with palbociclib and fulvestrant in women with HR+/HER2− ABC: updated exploratory analyses of PALOMA-3, a double-blind, phase III randomized study. Clin Cancer Res. 2022;28:3433-3442. https://doi.org/10.1158/1078-0432.CCR-22-0305.
|
[78] |
Slamon DJ, Neven P, Chia S, et al. Phase III randomized study of ribociclib and fulvestrant in hormone receptor–positive, human epidermal growth factor receptor 2–negative advanced breast cancer: MONALEESA-3. J Clin Oncol. 2018;36:2465-2472. https://doi.org/10.1200/JCO.2018.78.9909.
|
[79] |
Slamon DJ, Neven P, Chia S, et al. Overall survival with ribociclib plus fulvestrant in advanced breast cancer. N Engl J Med. 2020;382:514-524. https://doi.org/10.1056/NEJMoa1911149.
|
[80] |
Sledge GW, Toi M, Neven P, et al. MONARCH 2: abemaciclib in combination with fulvestrant in women with HR+/HER2− advanced breast cancer who had progressed while receiving endocrine therapy. J Clin Oncol. 2017;35:2875-2884. https://doi.org/10.1200/JCO.2017.73.7585.
|
[81] |
Sledge GW, Toi M, Neven P, et al. The Effect of abemaciclib plus fulvestrant on overall survival in hormone receptor–positive, ERBB2-negative breast cancer that progressed on endocrine therapy—MONARCH 2: a randomized clinical trial. JAMA Oncol. 2020;6:116. https://doi.org/10.1001/jamaoncol.2019.4782.
|
[82] |
Xu B, Zhang Q, Zhang P, et al. Dalpiciclib or placebo plus fulvestrant in hormone receptor-positive and HER2-negative advanced breast cancer: a randomized, phase 3 trial. Nat Med. 2021;27:1904-1909. https://doi.org/10.1038/s41591-021-01562-9.
|
[83] |
Dickler MN, Tolaney SM, Rugo HS, et al. MONARCH 1, a phase ii study of abemaciclib, a CDK4 and CDK6 inhibitor, as a single agent, in patients with refractory HR+/HER2− metastatic breast cancer. Clin Cancer Res. 2017;23:5218-5224. https://doi.org/10.1158/1078-0432.CCR-17-0754.
|
[84] |
Johnston SRD, Harbeck N, Hegg R, et al. Abemaciclib combined with endocrine therapy for the adjuvant treatment of HR+, HER2−, node-positive, high-risk, early breast cancer (monarchE). J Clin Oncol. 2020;38:3987-3998. https://doi.org/10.1200/JCO.20.02514.
|
[85] |
Johnston SRD, Toi M, O’Shaughnessy J, et al. Abemaciclib plus endocrine therapy for hormone receptor-positive, HER2-negative, node-positive, high-risk early breast cancer (monarchE): results from a preplanned interim analysis of a randomised, open-label, phase 3 trial. Lancet Oncol. 2023;24:77-90. https://doi.org/10.1016/S1470-2045(22)00694-5.
|
[86] |
Tan AR, Wright GS, Thummala AR, et al. Trilaciclib plus chemotherapy versus chemotherapy alone in patients with metastatic triple-negative breast cancer: a multicentre, randomised, open-label, phase 2 trial. Lancet Oncol. 2019;20:1587-1601. https://doi.org/10.1016/S1470-2045(19)30616-3.
|
[87] |
Weiss JM, Csoszi T, Maglakelidze M, et al. Myelopreservation with the CDK4/6 inhibitor trilaciclib in patients with small-cell lung cancer receiving first-line chemotherapy: a phase Ib/randomized phase II trial. Ann Oncol. 2019;30:1613-1621. https://doi.org/10.1093/annonc/mdz278.
|
[88] |
He S, Roberts PJ, Sorrentino JA, et al. Transient CDK4/6 inhibition protects hematopoietic stem cells from chemotherapy-induced exhaustion. Sci Transl Med. 2017;9:eaal3986. https://doi.org/10.1126/scitranslmed.aal3986.
|
[89] |
Pandey K, An H, Kim SK, et al. Molecular mechanisms of resistance to CDK4/6 inhibitors in breast cancer: a review. Int J Cancer. 2019;145:1179-1188. https://doi.org/10.1002/ijc.32020.
|
[90] |
Álvarez-Fernández M, Malumbres M. Mechanisms of sensitivity and resistance to CDK4/6 inhibition. Cancer Cell. 2020;37:514-529. https://doi.org/10.1016/j.ccell.2020.03.010.
|
[91] |
Guarducci C, Bonechi M, Boccalini G, et al. Mechanisms of resistance to CDK4/6 inhibitors in breast cancer and potential biomarkers of response. Breast Care. 2017;12:304-308. https://doi.org/10.1159/000484167.
|
[92] |
Wander SA, Cohen O, Gong X, et al. The genomic landscape of intrinsic and acquired resistance to cyclin-dependent kinase 4/6 inhibitors in patients with hormone receptor-positive metastatic breast cancer. Cancer Discov. 2020;10:1174-1193. https://doi.org/10.1158/2159-8290.CD-19-1390.
|
[93] |
Cai Z, Shi Q, Li Y, et al. LncRNA EILA promotes CDK4/6 inhibitor resistance in breast cancer by stabilizing cyclin E1 protein. Sci Adv. 2023;9:eadi3821. https://doi.org/10.1126/sciadv.adi3821.
|
[94] |
Navarro-Yepes J, Kettner NM, Rao X, et al. Abemaciclib is effective in palbociclib-resistant hormone receptor-positive metastatic breast cancers. Cancer Res. 2023;83:3264-3283.
|
[95] |
Pandey K, Park N, Park K-S, et al. Combined CDK2 and CDK4/6 inhibition overcomes palbociclib resistance in breast cancer by enhancing senescence. Cancers. 2020;12:3566. https://doi.org/10.3390/cancers12123566.
|
[96] |
Alves CL, Ehmsen S, Terp MG, et al. Co-targeting CDK4/6 and AKT with endocrine therapy prevents progression in CDK4/6 inhibitor and endocrine therapy-resistant breast cancer. Nat Commun. 2021;12:5112. https://doi.org/10.1038/s41467-021-25422-9.
|
[97] |
Al-Qasem AJ, Alves CL, Ehmsen S, et al. Co-targeting CDK2 and CDK4/6 overcomes resistance to aromatase and CDK4/6 inhibitors in ER+ breast cancer. NPJ Precis Oncol. 2022;6:68. https://doi.org/10.1038/s41698-022-00311-6.
|
[98] |
Zikry TM, Wolff SC, Ranek JS, et al. Cell cycle plasticity underlies fractional resistance to palbociclib in ER+/HER2− breast tumor cells. Proc Natl Acad Sci USA. 2024;121:e2309261121. https://doi.org/10.1073/pnas.2309261121.
|
[99] |
Herrera-Abreu MT, Palafox M, Asghar U, et al. Early adaptation and acquired resistance to CDK4/6 inhibition in estrogen receptor–positive breast cancer. Cancer Research. 2016;76:2301-2313.
|
[100] |
Costa C, Wang Y, Ly A, et al. PTEN loss mediates clinical cross-resistance to CDK4/6 and PI3Kα inhibitors in breast cancer. Cancer Discov. 2020;10:72-85. https://doi.org/10.1158/2159-8290.CD-18-0830.
|
[101] |
O’Leary B, Cutts RJ, Liu Y, et al. The genetic landscape and clonal evolution of breast cancer resistance to palbociclib plus fulvestrant in the PALOMA-3 trial. Cancer Discov. 2018;8:1390-1403. https://doi.org/10.1158/2159-8290.CD-18-0264.
|
[102] |
Jansen VM, Bhola NE, Bauer JA, et al. Kinome-wide RNA interference screen reveals a role for PDK1 in acquired resistance to CDK4/6 inhibition in ER-positive breast cancer. Cancer Res. 2017;77:2488-2499.
|
[103] |
Turner NC, Liu Y, Zhu Z, et al. Cyclin E1 expression and palbociclib efficacy in previously treated hormone receptor-positive metastatic breast cancer. J Clin Oncol. 2019;37:1169-1178. https://doi.org/10.1200/JCO.18.00925.
|
[104] |
Vijayaraghavan S, Karakas C, Doostan I, et al. CDK4/6 and autophagy inhibitors synergistically induce senescence in Rb positive cytoplasmic cyclin E negative cancers. Nat Commun. 2017;8:15916. https://doi.org/10.1038/ncomms15916.
|
[105] |
Freeman-Cook KD, Hoffman RL, Behenna DC, et al. Discovery of PF-06873600, a CDK2/4/6 inhibitor for the treatment of cancer. J Med Chem. 2021;64:9056-9077. https://doi.org/10.1021/acs.jmedchem.1c00159.
|
[106] |
Kramer C, Heinisch T, Fligge T, et al. A Consistent Dataset of Kinetic Solubilities for Early‐Phase Drug Discovery. ChemMedChem. 2009;4:1529-1536. https://doi.org/10.1002/cmdc.200900205.
|
[107] |
Waring MJ. Defining optimum lipophilicity and molecular weight ranges for drug candidates—molecular weight dependent lower logD limits based on permeability. Bioorgan Med Chem Lett. 2009;19:2844-2851. https://doi.org/10.1016/j.bmcl.2009.03.109.
|
[108] |
Johnson TW, Dress KR, Edwards M. Using the golden triangle to optimize clearance and oral absorption. Bioorgan Med Chem Lett. 2009;19:5560-5564. https://doi.org/10.1016/j.bmcl.2009.08.045.
|
[109] |
Ling Y, Hao ZY, Liang D, et al. The expanding role of pyridine and dihydropyridine scaffolds in drug design. Drug Des Devel Ther. 2021;15:4289-4338. https://doi.org/10.2147/DDDT.S329547.
|
[110] |
Freeman-Cook K, Hoffman RL, Miller N, et al. Expanding control of the tumor cell cycle with a CDK2/4/6 inhibitor. Cancer Cell. 2021;39:1404-1421. e11.
|
[111] |
Turner NC, Liu Y, Zhu Z, et al. Abstract CT039: Cyclin E1 (CCNE1) expression associates with benefit from palbociclib in metastatic breast cancer (MBC) in the PALOMA3 trial. Cancer Res. 2018;78:CT039. https://doi.org/10.1158/1538-7445.AM2018-CT039.
|
[112] |
Chen L, Yang N, Huang Y, et al. Development and validation of pharmaceutical care barriers scale in Chinese hospitals: a cross-sectional survey. Front Pharmacol. 2023;14:1194901. https://doi.org/10.3389/fphar.2023.1194901.
|
[113] |
Li J, Wang H, Hua Y, et al. Progress and challenges of the new conditional approval process in China: a pooled analysis from 2018 to 2021. Clin Ther. 2023;45:1111-1118. https://doi.org/10.1016/j.clinthera.2023.09.006.
|
[114] |
Ruan X, Wang Y, Zhou L, et al. Evaluation of untargeted metabolomic strategy for the discovery of biomarker of breast cancer. Front Pharmacol. 2022;13:894099. https://doi.org/10.3389/fphar.2022.894099.
|
[115] |
Wu H, Yao H, He C, et al. Molecular glues modulate protein functions by inducing protein aggregation: A promising therapeutic strategy of small molecules for disease treatment. Acta Pharm Sin B. 2022;12:3548-3566. https://doi.org/10.1016/j.apsb.2022.03.019.
|
[116] |
Zhou T, Sheng Y, Guan H. Cost-effectiveness of Vedolizumab in the treatment of moderate-to-Severe Crohn’s disease in China. Adv Ther. 2021;38:4233-4245. https://doi.org/10.1007/s12325-021-01806-7.
|
[117] |
Hua WQ, Lin J, Peng N, et al. Clinical development success rates for innovative drugs in China. Int J Pharmacol. 2022;18:1137-1150. https://doi.org/10.3923/ijp.2022.1137.1150.
|
[118] |
Yap TA, Elhaddad AM, Grisham RN, et al. First-in-human phase 1/2a study of a potent and novel CDK2-selective inhibitor PF-07104091 in patients (pts) with advanced solid tumors, enriched for CDK4/6 inhibitor resistant HR+/HER2- breast cancer. J Clin Oncol. 2023;41:3010. https://doi.org/10.1200/JCO.2023.41.16_suppl.3010.
|
[119] |
Patel MR, Juric D, Henick BS, et al. BLU-222, an oral, potent, and selective CDK2 inhibitor, in patients with advanced solid tumors: Phase I monotherapy dose escalation. J Clin Oncol. 2023;41:3095. https://doi.org/10.1200/JCO.2023.41.16_suppl.3095.
|
[120] |
Bisi JE, Sorrentino JA, Roberts PJ, et al. Preclinical characterization of G1T28: A novel CDK4/6 inhibitor for reduction of chemotherapy-induced myelosuppression. Mol Cancer Ther. 2016;15:783-793. https://doi.org/10.1158/1535-7163.MCT-15-0775.
|
[121] |
Dietrich C, Trub A, Ahn A, et al. INX-315, a selective CDK2 inhibitor, induces cell cycle arrest and senescence in solid tumors. Cancer Discov. 2024;14:446-467. https://doi.org/10.1158/2159-8290.CD-23-0954.
|
[122] |
Watts LP, Spencer SL. A highly anticipated selective therapeutic agent against CDK2: INX-315. Cancer Discov. 2024;14:386-388. https://doi.org/10.1158/2159-8290.CD-23-1537.
|
[123] |
Sokolsky A, Winterton S, Kennedy K, et al. Discovery of 5, 7-dihydro-6H-pyrrolo [2, 3-d] pyrimidin-6-ones as highly selective CDK2 inhibitors. ACS Med Chem Lett. 2022;13:1797-1804. https://doi.org/10.1021/acsmedchemlett.2c00408.
|
[124] |
Hummel JR, Xiao KJ, Yang JC, et al. Discovery of (4-pyrazolyl)-2-aminopyrimidines as potent and selective inhibitors of cyclin-dependent kinase 2. J Med Chem. 2024;67:3112-3126. https://doi.org/10.1021/acs.jmedchem.3c02287.
|
[125] |
Hardcastle IR, Arris CE, Bentley J, et al. N2-substituted O6-cyclohexylmethylguanine derivatives: potent inhibitors of cyclin-dependent kinases 1 and 2. J Med Chem. 2004;47:3710-3722. https://doi.org/10.1021/jm0311442.
|
[126] |
McInnes C, Wang S, Anderson S, et al. Structural determinants of CDK4 inhibition and design of selective ATP competitive inhibitors. Chem Biol. 2004;11:525-534. https://doi.org/10.1016/j.chembiol.2004.03.022.
|
[127] |
Lücking U, Jautelat R, Krüger M, et al. The lab oddity prevails: discovery of pan‐CDK inhibitor (R)‐S‐cyclopropyl‐S‐(4‐{[4‐{[(1R, 2R)‐2‐hydroxy‐1‐methylpropyl]oxy}‐5‐(trifluoromethyl)pyrimidin‐2‐yl]amino}phenyl)sulfoximide (BAY 1000394) for the treatment of cancer. ChemMedChem. 2013;8:1067-1085. https://doi.org/10.1002/cmdc.201300096.
|
[128] |
Stepan AF, Karki K, McDonald WS, et al. Metabolism-directed design of oxetane-containing arylsulfonamide derivatives as γ-secretase inhibitors. J Med Chem. 2011;54:7772-7783. https://doi.org/10.1021/jm200893p.
|
[129] |
Wang Y, Zhu J, Liu J (Jim), et al. Optimization beyond AMG 232: discovery and SAR of sulfonamides on a piperidinone scaffold as potent inhibitors of the MDM2-p53 protein–protein interaction. Bioorgan Med Chem Lett. 2014;24:3782-3785. https://doi.org/10.1016/j.bmcl.2014.06.073.
|
[130] |
Di Martino RMC, Maxwell BD, Pirali T. Deuterium in drug discovery: progress, opportunities and challenges. Nat Rev Drug Discov. 2023;22:562-584. https://doi.org/10.1038/s41573-023-00703-8.
|
[131] |
William AD, Lee ACH, Goh KC, et al. Discovery of kinase spectrum selective macrocycle (16 E )-14-methyl-20-oxa-5, 7, 14, 26-tetraazatetracyclo[19.3. 1.1(2, 6). 1(8, 12)]heptacosa-1(25), 2(26), 3, 5, 8(27), 9, 11, 16, 21, 23-decaene (SB1317/TG02), a potent inhibitor of cyclin-dependent kinases (CDKs), janus kinase 2 (JAK2), and fms-like tyrosine kinase-3 (FLT3) for the treatment of cancer. J Med Chem. 2012;55:169-196. https://doi.org/10.1021/jm201112g.
|
[132] |
Brasca MG, Amboldi N, Ballinari D, et al. Identification of N, 1,4,4-Tetramethyl-8-{[4-(4-methylpiperazin-1-yl)phenyl]amino}-4,5-dihydro-1 H-pyrazolo[4, 3-h ]quinazoline-3-carboxamide (PHA-848125), a potent, orally available cyclin dependent kinase inhibitor. J Med Chem. 2009;52:5152-5163. https://doi.org/10.1021/jm9006559.
|
[133] |
Yao H, Liu J, Xu S, et al. The structural modification of natural products for novel drug discovery. Expert Opin Drug Discov. 2017;12:121-140. https://doi.org/10.1080/17460441.2016.1272757.
|
[134] |
Das B, Baidya ATK, Mathew AT, et al. Structural modification aimed for improving solubility of lead compounds in early phase drug discovery. Bioorgan Med Chem. 2022;56:116614. https://doi.org/10.1016/j.bmc.2022.116614.
|
[135] |
Ishikawa M, Hashimoto Y. Improvement in aqueous solubility in small molecule drug discovery programs by disruption of molecular planarity and symmetry. J Med Chem. 2011;54:1539-1554. https://doi.org/10.1021/jm101356p.
|
[136] |
Lu Y, Luo Q, Jia X, et al. Multidisciplinary strategies to enhance therapeutic effects of flavonoids from Epimedii Folium: integration of herbal medicine, enzyme engineering, and nanotechnology. J Pharm Anal. 2023;13:239-254. https://doi.org/10.1016/j.jpha.2022.12.001.
|
[137] |
Musgrove EA, Caldon CE, Barraclough J, et al. Cyclin D as a therapeutic target in cancer. Nat Rev Cancer. 2011;11:558-572. https://doi.org/10.1038/nrc3090.
|
[138] |
VanArsdale T, Boshoff C, Arndt KT, et al. Molecular pathways: targeting the cyclin D–CDK4/6 axis for cancer treatment. Clin Cancer Res. 2015;21:2905-2910. https://doi.org/10.1158/1078-0432.CCR-14-0816.
|
[139] |
Roskoski R. Cyclin-dependent protein serine/threonine kinase inhibitors as anticancer drugs. Pharmacol Res. 2019;139:471-488. https://doi.org/10.1016/j.phrs.2018.11.035.
|
[140] |
Whittaker SR, Mallinger A, Workman P, et al. Inhibitors of cyclin-dependent kinases as cancer therapeutics. Pharmacol Therapeut. 2017;173:83-105. https://doi.org/10.1016/j.pharmthera.2017.02.008.
|
[141] |
Li M, Wang Y, Li M, et al. Integrins as attractive targets for cancer therapeutics. Acta Pharm Sin B. 2021;11:2726-2737. https://doi.org/10.1016/j.apsb.2021.01.004.
|
[142] |
Xin P, Xu X, Deng C, et al. The role of JAK/STAT signaling pathway and its inhibitors in diseases. Int Immunopharmacol. 2020;80:106210. https://doi.org/10.1016/j.intimp.2020.106210.
|
[143] |
Li X, Yang C, Wan H, et al. Discovery and development of pyrotinib: a novel irreversible EGFR/HER2 dual tyrosine kinase inhibitor with favorable safety profiles for the treatment of breast cancer. Eur J Pharm Sci. 2017;110:51-61. https://doi.org/10.1016/j.ejps.2017.01.021.
|
[144] |
Wang Y, Xiong B, Xing S, et al. Medicinal prospects of targeting tyrosinase: A feature review. Curr Med Chem. 2023;30:2638-2671. https://doi.org/10.2174/0929867329666220915123714.
|
[145] |
Gong L, Chen C, Liu X, et al. Hainanolide inhibits the progression of colon cancer via inducing the cell cycle arrest, cell apoptosis and activation of the MAPK signaling pathway. Toxicol Appl Pharmacol. 2022;454:116249. https://doi.org/10.1016/j.taap.2022.116249.
|
[146] |
Huang J, Wang X, Dong R, et al. Discovery of N-(4-(3-isopropyl-2-methyl-2H-indazol-5-yl)pyrimidin-2-yl)-4-(4-methylpiperazin-1-yl)quinazolin-7-amine as a novel, potent, and oral cyclin-dependent kinase inhibitor against haematological malignancies. J Med Chem. 2021;64:12548-12571. https://doi.org/10.1021/acs.jmedchem.1c00271.
|
[147] |
Yuan K, Kuang W, Chen W, et al. Discovery of novel and orally bioavailable CDK 4/6 inhibitors with high kinome selectivity, low toxicity and long-acting stability for the treatment of multiple myeloma. Eur J Med Chem. 2022;228:114024. https://doi.org/10.1016/j.ejmech.2021.114024.
|
[148] |
Yuan K, Min W, Wang X, et al. Discovery of novel and selective CDK4/6 inhibitors by pharmacophore and structure-based virtual screening. Future Med Chem. 2020;12:1121-1136. https://doi.org/10.4155/fmc-2020-0011.
|
[149] |
Yuan K, Wang X, Dong H, et al. Selective inhibition of CDK4/6: a safe and effective strategy for developing anticancer drugs. Acta Pharm Sin B. 2021;11:30-54. https://doi.org/10.1016/j.apsb.2020.05.001.
|
[150] |
Chen W, Ji M, Cheng H, et al. Discovery, optimization, and evaluation of selective CDK4/6 inhibitors for the treatment of breast cancer. J Med Chem. 2022;65:15102-15122. https://doi.org/10.1021/acs.jmedchem.2c00947.
|
[151] |
Chen P, Xu Y, Li X, et al. Development and strategies of CDK4/6 inhibitors. Future Med Chem. 2020;12:127-145. https://doi.org/10.4155/fmc-2019-0062.
|
[152] |
Wang Y, Zhi Y, Jin Q, et al. Discovery of 4-((7H-pyrrolo[2, 3-d]pyrimidin-4-yl)amino)-N-(4-((4-methylpiperazin-1-yl)methyl)phenyl)-1H-pyrazole-3-carboxamide (FN-1501), an FLT3- and CDK-kinase inhibitor with potentially high efficiency against acute myelocytic leukemia. J Med Chem. 2018;61:1499-1518. https://doi.org/10.1021/acs.jmedchem.7b01261.
|
[153] |
Li T, Weng T, Zuo M, et al. Recent progress of cyclin-dependent kinase inhibitors as potential anticancer agents. Future Med Chem. 2016;8:2047-2076. https://doi.org/10.4155/fmc-2016-0129.
|
[154] |
He L, Lu N, Dai Q, et al. Wogonin induced G1 cell cycle arrest by regulating Wnt/β-catenin signaling pathway and inactivating CDK8 in human colorectal cancer carcinoma cells. Toxicology. 2013;312:36-47. https://doi.org/10.1016/j.tox.2013.07.013.
|
[155] |
Zhu J, Cai Y, Kong M, et al. Design, synthesis, and biological evaluation for first GPX4 and CDK dual inhibitors. J Med Chem. 2024;67:2758-2776. https://doi.org/10.1021/acs.jmedchem.3c01890.
|
[156] |
Yang L, Zhang H, Hu R, et al. Wogonin induces G1 phase arrest through inhibiting CDK4 and cyclin D1 concomitant with an elevation in p21Cip1 in human cervical carcinoma HeLa cells. Biochem Cell Biol. 2009;87:933-942. https://doi.org/10.1139/O09-060.
|
[157] |
Lu Y, Sheng X, Liu C, et al. SERD-NHC-Au(I) complexes for dual targeting ER and TrxR to induce ICD in breast cancer. Pharmacol Res. 2023;190:106731. https://doi.org/10.1016/j.phrs.2023.106731.
|
[158] |
Wei Q, Xu Y, Liu W, et al. Cost-effectiveness of abemaciclib plus endocrine therapy in high-risk HR+/HER2–early breast cancer in China. Cost Eff Resour Alloc. 2023;21:91. https://doi.org/10.1186/s12962-023-00499-9.
|
[159] |
Zhang Y, Zhao G, Yu L, et al. Heat-shock protein 90α protects NME1 against degradation and suppresses metastasis of breast cancer. Br J Cancer. 2023;129:1679-1691. https://doi.org/10.1038/s41416-023-02435-3.
|
[160] |
Li J, Zhou J, Wang H, et al. Trends in disparities and transitions of treatment in patients with early breast cancer in China and the US, 2011 to 2021. JAMA Netw Open. 2023;6:e2321388. https://doi.org/10.1001/jamanetworkopen.2023.21388.
|
[161] |
Qiao YW, Yu G, Li GF. Overall survival benefit with sacituzumab govitecan in metastatic breast cancer: a post hoc interaction analyses of a randomized controlled trail. Clin Drug Investig. 2024;44:455-457. https://doi.org/10.1007/s40261-024-01367-x.
|
[162] |
Yang YH, Liu JW, Lu C, et al. CAR-T cell therapy for breast cancer: from basic research to clinical application. Int J Biol Sci. 2022;18:2609-2626. https://doi.org/10.7150/ijbs.70120.
|
[163] |
Wang T, You Q, Huang F, et al. Recent advances in selective estrogen receptor modulators for breast cancer. Mini Rev Med Chem. 2009;9:1191-1201. https://doi.org/10.2174/138955709789055207.
|
[164] |
Witkiewicz AK, Knudsen ES. Retinoblastoma tumor suppressor pathway in breast cancer: prognosis, precision medicine, and therapeutic interventions. Breast Cancer Res. 2014;16:3396.
|
[165] |
Guan Q, Gao Z, Chen Y, et al. Structural modification strategies of triazoles in anticancer drug development. Eur J Med Chem. 2024;275:116578. https://doi.org/10.1016/j.ejmech.2024.116578.
|
[166] |
Dong RF, Zhu ML, Liu MM, et al. EGFR mutation mediates resistance to EGFR tyrosine kinase inhibitors in NSCLC: from molecular mechanisms to clinical research. Pharmacol Res. 2021;167:105583. https://doi.org/10.1016/j.phrs.2021.105583.
|
[167] |
Liu Y, Chen Y, Jiang J, et al. Development of highly potent and specific AKR1C3 inhibitors to restore the chemosensitivity of drug-resistant breast cancer. Eur J Med Chem. 2023;247:115013. https://doi.org/10.1016/j.ejmech.2022.115013.
|
[168] |
Li Y, Liu R, Han X, et al. PLAGL2 increases adriamycin resistance and EMT in breast cancer cells by activating the Wnt pathway. Genes Genom. 2023;45:49-57. https://doi.org/10.1007/s13258-022-01330-0.
|
[169] |
Yang J, Yu YC, Wang ZX, et al. Research strategies of small molecules as chemotherapeutics to overcome multiple myeloma resistance. Eur J Med Chem. 2024;271:116435. https://doi.org/10.1016/j.ejmech.2024.116435.
|
[170] |
Qin M, Xin Y, Bian Y, et al. Phosphorylation-induced ubiquitination and degradation of PXR through CDK2-TRIM21 axis. Cells. 2022;11:264. https://doi.org/10.3390/cells11020264.
|
[171] |
Qian X, Dai X, Luo L, et al. An interpretable multitask framework BiLAT enables accurate prediction of cyclin-dependent protein kinase inhibitors. J Chem Inf Model. 2023;63:3350-3368. https://doi.org/10.1021/acs.jcim.3c00473.
|
[172] |
Tadesse S, Anshabo AT, Portman N, et al. Targeting CDK2 in cancer: challenges and opportunities for therapy. Drug Discov Today. 2020;25:406-413. https://doi.org/10.1016/j.drudis.2019.12.001.
|
[173] |
Arora M, Moser J, Hoffman TE, et al. Rapid adaptation to CDK2 inhibition exposes intrinsic cell-cycle plasticity. Cell. 2023;186:2628-2643. e21.
|
[174] |
Cai W, Tong R, Sun Y, et al. Comparative efficacy of five approved Janus kinase inhibitors as monotherapy and combination therapy in patients with moderate-to-severe active rheumatoid arthritis: a systematic review and network meta-analysis of randomized controlled trials. Front Pharmacol. 2024;15:1387585. https://doi.org/10.3389/fphar.2024.1387585.
|
[175] |
Roy D, Sheng GY, Herve S, et al. Interplay between cancer cell cycle and metabolism: challenges, targets and therapeutic opportunities. Biomed Pharmacother. 2017;89:288-296. https://doi.org/10.1016/j.biopha.2017.01.019.
|
[176] |
Xiong S, Song K, Xiang H, et al. Dual-target inhibitors based on ERα: Novel therapeutic approaches for endocrine resistant breast cancer. Eur J Med Chem. 2024;270:116393. https://doi.org/10.1016/j.ejmech.2024.116393.
|
[177] |
Chen X, He R, Chen X, et al. Optimizing dose-schedule regimens with bayesian adaptive designs: opportunities and challenges. Front Pharmacol. 2023;14:1261312. https://doi.org/10.3389/fphar.2023.1261312.
|
[178] |
Sun Y, Yu X, Wang X, et al. Bispecific antibodies in cancer therapy: target selection and regulatory requirements. Acta Pharm Sin B. 2023;13:3583-3597. https://doi.org/10.1016/j.apsb.2023.05.023.
|
[179] |
Xiang Y, Liu X, Wang Y, et al. Mechanisms of resistance to targeted therapy and immunotherapy in non-small cell lung cancer: promising strategies to overcoming challenges. Front Immunol. 2024;15:1366260. https://doi.org/10.3389/fimmu.2024.1366260.
|
[180] |
Li Y, Li X, Zhu M, et al. Development of a physiologically based pharmacokinetic population model for diabetic patients and its application to understand disease-drug–drug interactions. Clin Pharmacokinet. 2024;63:831-845 https://doi.org/10.1007/s40262-024-01383-2.
|
[181] |
Chen Y, Xu S, Ren S, et al. Design of a targeted dual drug delivery system for boosting the efficacy of photoimmunotherapy against melanoma proliferation and metastasis. J Adv Res. 2024;S2090-1232(24)00207-8. https://doi.org/10.1016/j.jare.2024.05.017.
|
[182] |
Krebs MG, Lord S, Kenny L, et al. 230MO First in human, modular study of samuraciclib (CT7001), a first-in-class, oral, selective inhibitor of CDK7, in patients with advanced solid malignancies. Ann Oncol. 2021;32:S458.
|
[183] |
Coombes C, Howell SJ, Krebs MG, et al. Abstract GS3-10: study of samuraciclib (CT7001), a first-in-class, oral, selective inhibitor of CDK7, in combination with fulvestrant in patients with advanced hormone receptor positive HER2 negative breast cancer (HR + BC). Cancer Res. 2022;82:GS3.
|
[184] |
Coombes RC, Howell S, Lord SR, et al. Dose escalation and expansion cohorts in patients with advanced breast cancer in a Phase I study of the CDK7-inhibitor samuraciclib. Nat Commun. 2023;14:4444. https://doi.org/10.1038/s41467-023-40061-y.
|
[185] |
Wang M, Wang T, Zhang X, et al. Cyclin-dependent kinase 7 inhibitors in cancer therapy. Future Med Chem. 2020;12:813-833. https://doi.org/10.4155/fmc-2019-0334.
|
[186] |
Wander SA, Han HS, Zangardi ML, et al. Clinical outcomes with abemaciclib after prior CDK4/6 inhibitor progression in breast cancer: A multicenter experience. J Natl Compr Canc Netw. 2021;24:1-8.
|
[187] |
Yao T, Xiao H, Wang H, et al. Recent advances in PROTACs for drug targeted protein research. Int J Mol Sci. 2022;23:10328. https://doi.org/10.3390/ijms231810328.
|
[188] |
Wang Y, Jiang X, Feng F, et al. Degradation of proteins by PROTACs and other strategies. Acta Pharm Sin B. 2020;10:207-238. https://doi.org/10.1016/j.apsb.2019.08.001.
|
[189] |
Xie S, Zhu J, Li J, et al. Small-molecule hydrophobic tagging: a promising strategy of druglike technology for targeted protein degradation. J Med Chem. 2023;66:10917-10933. https://doi.org/10.1021/acs.jmedchem.3c00736.
|
[190] |
Ma S, Ji J, Tong Y, et al. Non-small molecule PROTACs (NSM-PROTACs): protein degradation kaleidoscope. Acta Pharm Sin B. 2022;12:2990-3005. https://doi.org/10.1016/j.apsb.2022.02.022.
|
[191] |
Hua L, Zhang Q, Zhu X, et al. Beyond proteolysis-targeting chimeric molecules: designing heterobifunctional molecules based on functional effectors. J Med Chem. 2022;65:8091-8112. https://doi.org/10.1021/acs.jmedchem.2c00316.
|
[192] |
Xue X, Li Q, Zhang P, et al. PET/NIR fluorescence bimodal imaging for targeted tumor detection. Mol Pharm. 2023;20:6262-6071. https://doi.org/10.1021/acs.molpharmaceut.3c00660.
|
[193] |
Wang YW, Lan L, Wang M, et al. PROTACS: A technology with a gold rush-like atmosphere. Eur J Med Chem. 2023;247:115037. https://doi.org/10.1016/j.ejmech.2022.115037.
|
[194] |
Zhong Y, Chi F, Wu H, et al. Emerging targeted protein degradation tools for innovative drug discovery: From classical PROTACs to the novel and beyond. Eur J Med Chem. 2022;231:114142. https://doi.org/10.1016/j.ejmech.2022.114142.
|
[195] |
Dong X, Sun R, Wang J, et al. Glutathione S-transferases P1-mediated interleukin-6 in tumor-associated macrophages augments drug-resistance in MCF-7 breast cancer. Biochem Pharmacol. 2020;182:114289. https://doi.org/10.1016/j.bcp.2020.114289.
|
[196] |
Yang N, Kong B, Zhu Z, et al. Recent advances in targeted protein degraders as potential therapeutic agents. Mol Divers. 2024;28:309-333. https://doi.org/10.1007/s11030-023-10606-w.
|
[197] |
Zhu P, Zhang J, Yang Y, et al. Design, synthesis and biological evaluation of isoxazole-containing biphenyl derivatives as small-molecule inhibitors targeting the programmed cell death-1/ programmed cell death-ligand 1 immune checkpoint. Mol Divers. 2022;26:245-264. https://doi.org/10.1007/s11030-021-10208-4.
|
[198] |
Zhou QQ, Xiao HT, Yang F, et al. Advancing targeted protein degradation for metabolic diseases therapy. Pharmacol Res. 2023;188:106627. https://doi.org/10.1016/j.phrs.2022.106627.
|
[199] |
Sang H, Liu J, Zhou F, et al. Target-responsive subcellular catabolism analysis for early-stage antibody–drug conjugates screening and assessment. Acta Pharm Sin B. 2021;11:4020-4031. https://doi.org/10.1016/j.apsb.2021.05.024.
|
[200] |
Wang Y, Rui M, Guan X, et al. Cost-effectiveness analysis of abemaciclib plus fulvestrant in the second-line treatment of women with HR+/HER2– advanced or metastatic breast cancer: A US payer perspective. Front Med. 2021;8:658747. https://doi.org/10.3389/fmed.2021.658747.
|
[201] |
Zhang K, Li J, Xin X, et al. Dual targeting of cancer cells and MMPs with self-assembly hybrid nanoparticles for combination therapy in combating cancer. Pharmaceutics. 2021;13:1990. https://doi.org/10.3390/pharmaceutics13121990.
|
[202] |
Shao H, Zhao M, Guan AJ, et al. A network meta-analysis of efficacy and safety for first-line and second/further-line therapies in postmenopausal women with hormone receptor-positive, HER2-negative, advanced breast cancer. BMC Med. 2024;22:13. https://doi.org/10.1186/s12916-023-03238-2.
|
[203] |
Wang L, Xu X, Jiang Z, et al. Modulation of protein fate decision by small molecules: targeting molecular chaperone machinery. Acta Pharm Sin B. 2020;10:1904-1925. https://doi.org/10.1016/j.apsb.2020.01.018.
|
[204] |
Yu B, Du Z, Zhang Y, et al. Small-molecule degraders of cyclin-dependent kinase protein: a review. Future Med Chem. 2022;14:167-185. https://doi.org/10.4155/fmc-2021-0154.
|
[205] |
Xing S, Li Q, Xiong B, et al. Structure and therapeutic uses of butyrylcholinesterase: Application in detoxification, Alzheimer’s disease, and fat metabolism. Med Res Rev. 2021;41:858-901. https://doi.org/10.1002/med.21745.
|
[206] |
Xiao K, Liu F, Liu J, et al. The effect of metformin on lung cancer risk and survival in patients with type 2 diabetes mellitus: a meta‐analysis. J Clin Pharm Ther. 2020;45:783-792. https://doi.org/10.1111/jcpt.13167.
|
[207] |
He Q, Zhao X, Wu D, et al. Hydrophobic tag-based protein degradation: development, opportunity and challenge. Eur J Med Chem. 2023;260:115741. https://doi.org/10.1016/j.ejmech.2023.115741.
|
[208] |
Dorjsuren B, Chaurasiya B, Ye Z, et al. Cetuximab-coated thermo-sensitive liposomes loaded with magnetic nanoparticles and doxorubicin for targeted EGFR-expressing breast cancer combined therapy. Int J Nanomedicine. 2020;15:8201-8215. https://doi.org/10.2147/IJN.S261671.
|
[209] |
Zheng M, Zhang XY, Chen W, et al. Molecules inducing specific cyclin-dependent kinase degradation and their possible use in cancer therapy. Future Med Chem. 2024;16:369-388. https://doi.org/10.4155/fmc-2023-0259.
|
[210] |
Ha S, Luo G, Xiang H. A comprehensive overview of small-molecule androgen receptor degraders: recent progress and future perspectives. J Med Chem. 2022;65:16128-16154. https://doi.org/10.1021/acs.jmedchem.2c01487.
|
[211] |
Tsang WP, Zhang F, He Q, et al. Icaritin enhances mESC self-renewal through upregulating core pluripotency transcription factors mediated by ERα. Sci Rep. 2017;7:40894. https://doi.org/10.1038/srep40894.
|
[212] |
Luo P, An Y, He J, et al. Icaritin with autophagy/mitophagy inhibitors synergistically enhances anticancer efficacy and apoptotic effects through PINK1/Parkin-mediated mitophagy in hepatocellular carcinoma. Cancer Lett. 2024;587:216621. https://doi.org/10.1016/j.canlet.2024.216621.
|
Drug | NCT number | Stage | Disease |
Palbociclib | NCT02310243 | I/II | Acute leukemias |
Palbociclib | NCT01356628 | II | Liver cancer |
Palbociclib | NCT02905318 | II | Prostate cancer |
Ribociclib/Everolimus | NCT02985125 | I/II | Pancreatic adenocarcinoma |
Ribociclib | NCT03096912 | II | Liposarcoma |
Ribociclib/Letrozole | NCT03673124 | II | Ovarian cancer |
Abemaciclib | NCT04750928 | I/II | Atypical neurofibromas |
Abemaciclib | NCT03220646 | II | Brain tumor |
Abemaciclib | NCT04967521 | III | Dedifferentiated liposarcoma |
Abemaciclib | NCT04010357 | II | Small-cell lung cancer |
Name | Structure | Company | Stage |
PF-06873600 | ![]() |
Pfizer | Discontinued |
NUV-422 | ![]() |
Nuvation Bio | Discontinued |
RGT-419B | Not available | Regor | Phase I |
SCR-8079 | Not available | Simcere | Preclinical |
Name | Structure | Company | Datea |
PF07104091 | ![]() |
Pfizer | 2020-09-16 |
BLU-222 | ![]() |
Blueprint Medicine | 2022-04-07 |
INCB123667 | Not available | Incyte | 2022-07-05 |
INX-315 | ![]() |
Incyclix Bio | 2023-03-28 |
ARTS-021 | Not available | Allorion Therapeutics | 2023-08-30 |
AZD8421 | ![]() |
AstraZeneca | 2023-12-05 |
NKT-3447 | Not available | NiKang Therapeutics | 2024-02-23 |
BG-68501 | Not available | Beigene | 2024-02-28 |
aThe actual date on which the first participant was enrolled in the clinical study. |